Transformer oil investigations in the OELCHECK laboratory
Depending on the all-inclusive analysis kit selected, used transformer oils are analyzed using the following common test methods:
Breakdown voltage, viscosity, acid number, density, color number, oxidation, elements, purity class, MPC test, water.
In addition, OELCHECK analyzes insulating and transformer oils using the three methods presented in this article.
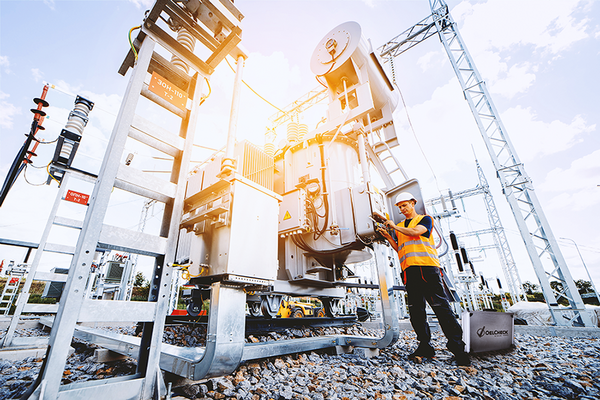
Table of contents
Dissolved Gas Analysis
Test standard: ASTM D3612-02, VDE0370-9:1994-06, VDE0370-7:1999-12
Measuring range: 0-10,000
Unit: µl/l (ppm)
Sample volume: 5 ml
Determined:
Dissolved gases in transformer oils
H2, O2, N2, CO2, CO, CH4, C2H6, C2H4, C2H2
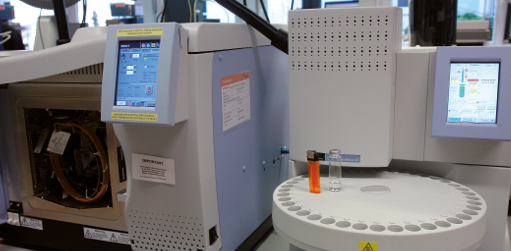
The analysis of gases present in the transformer oil in dissolved form is carried out with a PerkinElmer Clarus 680 with a TurboMatrix 40 Headspace Sampler, specially developed for this purpose.
This analytical procedure is used to evaluate defects in electrical equipment with the aim of initiating countermeasures in good time. Dissolved gas analysis is the most frequently performed method for monitoring transformers. It offers many advantages and not only for the detection of defects:
- Reliable information on the condition of a transformer
- Timely recognition of defects
- Condition monitoring of new and repaired systems
- Scope for planning of measures.
The basic principle of the dissolved gas analysis is based on the capacity of the insulating oil to dissolve not only air from the atmosphere, but also cracked gases. These evolve not only from the natural ageing of oil and insulation materials, but above all when thermal or electrical defects occur in transformers. The extent of a defect and its increase over time (trend analysis) can be concluded from the quantity of dissolved gases in the respective oil.
The cracked gases, also termed pollutant gases, evolve as a result of high-energy electrical discharges as well as partial discharges. However, local overheating can also cause the evolution of pollutant gases. If pollutant gases are discovered in the analysis of the transformer oil, this indicates sources of defects in the transformer.
These dissolved gases can only be measured with a gas chromatograph (GC). In order to ensure correct measurement results, the oil sample must not come into contact with atmospheric oxygen. For this reason, as soon as the oil is sampled, it is poured into a 20 ml gas-tight glass syringe. A crimped head space bottle is flushed with argon to remove air. From the syringe, 5 ml of sample are injected directly through a canula via the 3-way stopcock. In the sampler, the gases are expelled from the sample at 80°C and are then gradually heated in a GC oven up to 200°C. The escaping gases are flushed with the aid of argon (carrier gas) through a „column“ (about 10 m long, wrapped small glass tubes). Depending on the structure of the respective gases, these remain on the surface of the column for different lengths of time. In order to ensure clean separation, two different columns are used. Detectors recognise the individual components and record corresponding chromatograms. The larger the area under a peak, the greater the proportion of the corresponding component.
The following pollutant gases are determined using the chromatograph: H2 (hydrogen), O2 (oxygen), N2 (nitrogen), CH4 (methane), CO (carbon monoxide), CO2 (carbon dioxide), C2H6 (ethane), C2H4 (ethene/ethylene), C2H2 (ethyne/acetylene).
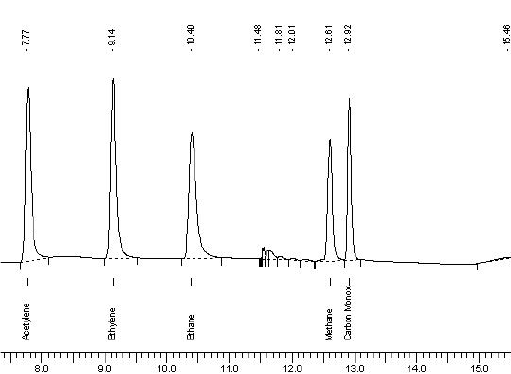
In the laboratory report, the respective individual values and their sum are indicated in µl/litre and/or ppm. An analysis is recommended at regular intervals, since by observing a trend, it is possible to react to changes in good time.
Depending on the intensity of the individual gases, differentiation is made between different defects. Different conclusions can be drawn from the respective defects with regard to the consequences for the oil or paper insulation. These values and their diagnoses are noted in the laboratory report in compliance with evaluation procedures defined in the VDE standard.
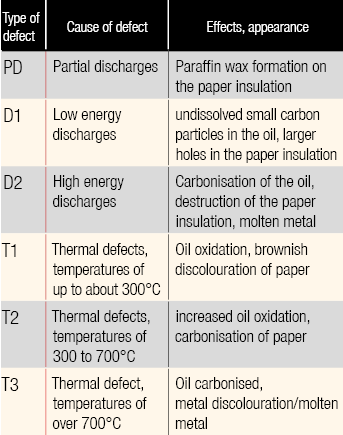
In addition to the values for pollutant gases, the Duval triangle is illustrated in the laboratory report. This allows the visualisation of the type of defect present. At increased concentrations, a summary statement can be generated using this tool.
Example: in a DGA analysis, the following percentages were calculated based on the ppm values: Ethylene (C2H4) = 36.8%, acetylene (C2H2) = 21.1%, methane (CH4) = 42.1%.
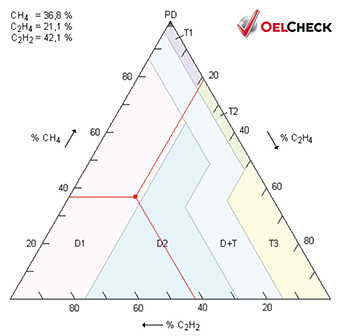
These values are entered into an isosceles Duval triangle parallel to the sides. The point at which the three lines intersect lies in a field with the types of defect listed in the table. In this way, a conclusion about the type of defect present in the case of example = D1 can be made.
Reference values for which a more frequent analysis is advisable, if exceeded:
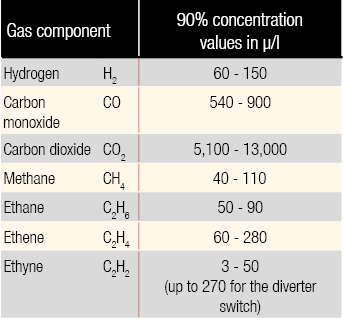
The dielectric dissipation factor
Test standard: IEC 60247, ASTM D924
Measuring range: tan δ up to 1x10-6
Sample volume: 40 ml
Determined:
Dissipation factor tan δ relative dielectric constant εr.
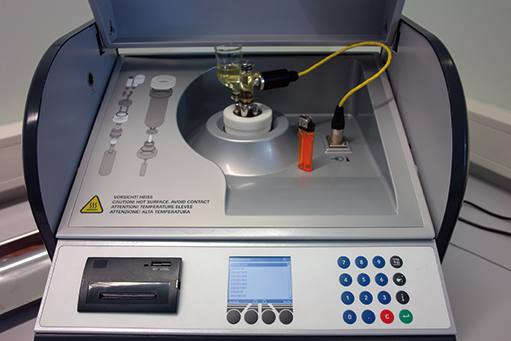
The dielectric dissipation factor tan δ measured with a Baur DTL-C provides information on the extent of dielectric losses in the transformer oil occurring during operation. It is defined as the ratio between the active and reactive currents flowing in a measurement circuit.
In determining the dielectric dissipation factor, it is assumed that current and voltage in an alternating current circuit are subject to a phase shift. This phase change is due to the fact that molecules in the insulation oil can no longer align with the alternating electric field. In order to define the tan δ, the measuring cell of the device is filled with 40 ml of oil and heated to a standard 90°C. A test voltage of 2,000 V at a frequency of 50 Hz is applied across the two electrodes of the measuring cell, which are arranged at a distance of 2 mm. The dielectric dissipation factor is dimensionless. It is noted in the laboratory report as tan δ, with a corresponding numerical value.
When the oil ages, polar components occur in the oil, leading to a phase displacement and in turn to dielectric losses in the insulating oil. Impurities such as water, dissolved insulating resin and paper can also have polar effects and thereby influence the dielectric dissipation factor. The tan δ is also significantly influenced by the temperature-dependent viscosity, and with it, the size of the molecules, in addition to the ageing of the oil and the impurities. The sum of these changes is expressed as tan δ . At the same time, the dielectric constant εr required for the design of most on-line oil sensors is also defined during measurement.
A sharply negative trend of the dissipation factor indicates unwanted heat generation within the insulating liquid and the fixed insulation, which can ultimately lead to thermal breakdown. For this reason, this test is highly significant for assessment of the condition of the complex arrangement of a transformer.
Value ranges for tan δ
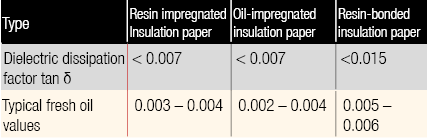
Interface tension
Test standard: ASTM D971–99, DIN 53914
Measuring range: 0 - 300 mN/m
Unit: mN/m (milliNewton/Meter)
Sample volume: 20 ml
Determined:
The interface tension between water and oil.
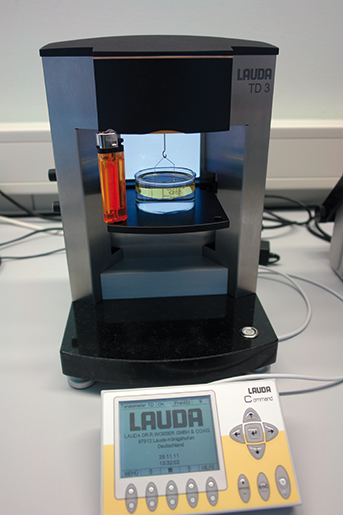
During the oxidation and ageing of oils subject to heavy demands or which are in use for several years, insoluble polar constituents are formed. Interface tension can detect these in the oil. In this way, it provides information on the ageing and remaining useful life of the oil.
In order to determine this with the Lauda TD 3 tensiometer, a testing cylinder is filled with 20 ml each of distilled water and the transformer oil to be tested. Due to the differences in density, two phases are rapidly formed, with the heavier water forming the lower phase and the insulating oil floating above. Fresh transformer oil is non-polar. Polar components have formed in the used oil, which accumulate in the region of the barrier layer of water (polar) and oil (non-polar). The interface tension of the water/oil phases is influenced by the interaction of the ageing products with the water. The Lecomte Du Noüy ring, secured by a thread to a scale, is lowered into the water-oil mixture. By lowering the cylinder, the ring is first drawn through the water and then through the interface into the oil. The force occurring during phase change at the interface is measured.
The interface tension is specified in mN/m in the laboratory report. If its value has fallen sharply by comparison with fresh oil or the previous sample, then ageing of the oil has occurred. The engineer will then recommend appropriate diagnostic countermeasures, such as regeneration or an
oil change.
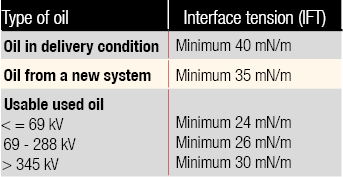
OELCHECKER Winter 2011, page 7-8