Hydraulic fluids and problematic mixtures
Hydraulic oils account for over 10 % of all lubricant consumption, placing them in second position behind engine oils at 40 %. They are used to transfer forces and movement, often in quantities of many hundreds of litres and at oil change intervals of several thousand operating hours. They are found primarily in construction and agricultural machinery, injection moulding machines, hydraulic presses, turbine regulator systems, lifts, aeroplanes, loading machinery, and many other pieces of industrial equipment. All of these are dependent on high-performance hydraulic oils. If hydraulic fluids are mixed together, on the other hand, all sorts of problems can arise ...
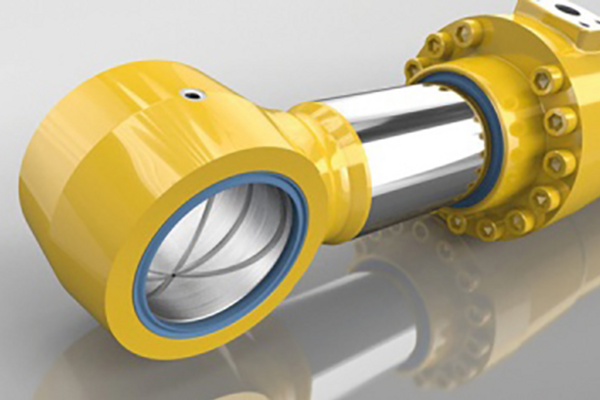
Table of contents
- Advantages of synthetic oils
-
Mixing hydraulic oils
- Note:
Please contact our OELCHECK tribologists (ta(at)oelcheck.de, phone +49 8034 9047-250) before ordering a compatibility analysis or if you suspect mixing. They will be happy to assist you in selecting the appropriate scope of analysis for your problem! - Compatibility analysis for hydraulic fluids
- Note:
- Checking hydraulic fluids and their compatibility
- Air release time
- Foaming tendency
- Water separation
- Filterability
- Conclusion
Hydraulic fluids perform all sorts of different tasks:
- transferring forces and movement
- control functions
- lubricating moving parts, including protecting against wear and reducing friction
- protecting against corrosion
- cooling (temperatures > 100°C possible)
- dampening vibrations
As hydraulic systems are optimised, so the requirements placed on hydraulic fluids increase. Systems are becoming ever more compact and designed for smaller oil quantities. The oil consequently spends less time in the oil container, and has less time to cool to the ideal mineral oil temperature of less than 60°C. At a temperature of 10°C higher, however, the increased oxidation tendency of mineral oils means that oil service life is halved. Due to oxidation, a mineral oil-based hydraulic oil that is changed after 10,000 operating hours at 60°C must be changed after only 2,500 operating hours at 80°C. As such, the oxidation or ageing stability of oils is becoming ever more relevant. Temperatures are not only rising because the oil quantities are falling, but also because pressures are increasing. If only a few years ago oil suited to 400 bar of pressure was needed for hydraulic engines and cylinders, today it is not unusual to encounter demands for oil suited to over 600 bar of pressure. With improved base oils and corresponding additives, modern hydraulic fluids are mastering these higher temperature requirements, as well as increased mechanical strains, and are contributing decisively to the continuous operability of the systems involved. These high demands can often only be met by using synthetic multi-purpose hydraulic oils, which are more suited to long-term use. The advantages of synthetic oils over conventional mineral oils can also be clearly proven using oil analysis.
Advantages of synthetic oils
Until the turn of the millennium, hydraulic oils were for the most part simply refined and mineral oilbased, with minimal additives. Above all, these were economical. Today, the only mineral oil-based hydraulic fluids still used are mostly HLP oils. Their base oils are oxidation-resistant hydrocracked products (CH) produced using hydrogenation, a hydrogen treatment carried out in the refinery. The Extreme Pressure (EP) and Anti-Wear (AW) active ingredients added in most cases contain a combination of zinc, phosphorus, and sulphur.
In addition to hydrocracked oils, which are still very inexpensive, today fully synthetically produced fluids are used. As base oils, these contain poly-alpha olefins (PAOs), saturated or unsaturated ester oils, or polyglycols (PAGs). Pure synthetic oils can remain in use for far longer than previously used products. Their molecular structure exhibits a lower reactivity with oxygen at higher operating temperatures. These oils also contain modern antioxidants based on phenols, amines, or salicylates, which serve to further improve service life. Synthetic base oils offer the advantage of better viscosity-temperature (VT) behaviour. These oils are thinner at low temperatures and thicker at higher temperatures than conventional mineral oils. Hydraulic systems filled with these are easier to start, and demonstrate smaller energy losses at high temperatures. A natural viscosity index (VI) as high as in excess of 200 instead of 95 eliminates the need for multigrade additives, which, as non shear-stable additives, can result in the formation of reaction products. These in turn cause deposits and lead to filtration problems.
Synthetic oils therefore minimise the risk of deposits and deliver a better filterability. Their consistently high VI improves cold start behaviour, ensures a stable viscosity level over a wide temperature range, and simplifies control. Flow and churning losses are reduced. The disadvantages of synthetic oils include their price, which is up to 10 times higher, reduced compatibility with one other, and problematic behaviour with respect to seals, tubing, and coats of paint.
Mixing hydraulic oils
Note:
Please contact our OELCHECK tribologists (ta(at)oelcheck.de, phone +49 8034 9047-250) before ordering a compatibility analysis or if you suspect mixing. They will be happy to assist you in selecting the appropriate scope of analysis for your problem!
Although lubricants are usually miscible (with the exception of PAGs) – especially if they share the same nominal viscosity and are designed for the same purpose – they are not always compatible with each other. In particular, when zinc-free and zinc-containing HLP mineral oils or PAO or ester synthetic oils are mixed with mineral oils or with synthetic oils from another producer, important characteristics such as air release time, water separation, foaming tendency, filterability, and seal compatibility can be negatively affected. Mixing of different hydraulic fluids, as happens for example in the case of construction machinery through the exchanging of different attachments on a daily basis, cannot always be avoided. In the event of difficulties following dismantling or an oil change, questions concerning the compatibility of two hydraulic fluids often arise. OELCHECK tribologists are able to determine the cause of the Chemical structure of mineral oil Chemical structure of synthetic oil 6 difficulties more easily if they have access to the following information:
- exact oil type, with information on base oil types and additives
- working temperatures and pressures
- machine designation and components in use
- usage times of the fluids
Compatibility analysis for hydraulic fluids
A standard analysis, which is conducted using an analysis kit 2 or 4, is usually sufficient to provide an indication of the problem‘s cause. Sometimes, however, it is necessary to carry out an explicit compatibility analysis.
For this, the lab usually requires five samples: A fresh oil sample of the two hydraulic fluids used, as well as three mixtures in the ratios 50:50, 95:5, and 5:95. The Sample Information Form must be marked „compatibility test“.
The OELCHECK lab will then begin by carrying out the same tests conducted using analysis kit 2. Air release time, foaming tendency, water separation, and water content are also determined. In addition, experienced OELCHECK tribologists also assess whether the oils are compatible with one another, and/or what problems may arise from mixing them.
Checking hydraulic fluids and their compatibility
The analysis set 2 is usually recommended for the routine monitoring of mineral oil-based hydraulic oils from plants with filling volumes up to approx. 1,000 liters.
The scope of analysis of Set 2 includes:
- Wear metals: iron, chromium, tin, aluminum, nickel, copper, lead, manganese.
- Magnetizable iron: PQ index ƒ Additives: zinc, phosphorus, sulfur, silicon (antifoam), calcium, magnesium, barium, boron, molybdenum
- Impurities: Silicon (dust), potassium, sodium, lithium (grease), water (above 0.1% measured by IR spectroscopy and by means of a "splash sample").
- Oil condition: viscosity at 40 °C and 100 °C, viscosity index, oxidation by FT-IR and optical impression (diesel effect)
- Oil cleanliness: cleanliness class according to ISO 4406 (particles >4 µ, 10µ and 14 µ).
For the evaluation of larger oil fillings or when using synthetic oils, the analysis set 4 should be used.
The scope of analysis of Set 4 additionally includes:
- the exact water content in ppm
- the acid content as Acid Number (AN)
With additional "individual tests" (note: this requires a further quantity of oil), the compatibility can be assessed even better. Ultimately, however, close observation of the system is always decisive, as the actual conditions cannot be reproduced in detail with a laboratory analysis.
Air release time
All oils contain air – fresh hydraulic oil contains approximately 9 vol. %. The amount of air that an oil is able to absorb is influenced among other factors by the oil temperature, oil type, viscosity, system pressure, additives, and mixture. The dissolved air itself does not normally cause any disruptions to operation. On the other hand, massive problems can be caused by air release time that is increased in comparison to fresh oil by impurities and oxidation products. An increased percentage of undissolved air in the form of mostly visible air bubbles can lead to the „springing“ of the oil charge. Exact control and positioning is then no longer possible. In the vicinity of the hydraulic pump – the component with the highest temperature in the circuit – increased quantities of undissolved air become separated. This can lead via implosion to cavitation, and in turn to the removal throughout the circuit of material from the components of hydraulic pumps or engines. In addition, the oxygen-containing air bubbles can also cause the „diesel effect“, which is often noticeable in its advanced stage from the darkening of the oil colour by carbon particles (soot). This effect arises when the oxygen present in the surplus air bubbles is so strongly compressed together with the surrounding carbons of the oil that a spontaneous combustion of this mixture occurs. The relatively small oxygen content results in incomplete combustion and the formation of soot particles, as found in a diesel engine. This introduces fine black particles into the oil which can also be found in the filter. Incidentally, air release time cannot be improved with additives – they can only be made worse.
Foaming tendency
Foam occurs on the surface of oil charges when air bubbles of a few µ to 1 mm in size separate from within the interior of an oil tank charge, rise to the surface, and then fail to immediately burst or disintegrate. These then form a stable foam layer on the oil surface. Foam formation is influenced by the surface tension of the oil, by operating temperature and its effects on viscosity, and by the air intake method. Mixtures of oils of different compositions, impurities, and also oil oxidation can cause an oil to foam more strongly. Oils are often designated as miscible. This does not mean, however, that oils are always truly „compatible“ with one another. When a PAO-based oil is mixed with a detergent HLP hydraulic oil, or a saturated ester-based bio-oil is mixed with an unsaturated ester, the surface tension of the fluids is altered. It is also possible for differently formulated oils in which foaming tendency has been improved by the addition of silicone-containing additives to change their foaming tendency when mixed, which can lead to the emergence of foam from all possible openings. For most systems, a foam layer up to 5 cm high presents no issue. Problems arise, however, when a sudden increase in foaming tendency is observed. In these cases, the surface foam can act as an insulating carpet, reducing heat dissipation, or flow from openings in the system. In addition to polluting the environment, the resulting loss of oil can lead to insufficient lubrication. The more active EP and AW ingredients an oil contains as additives, the more likely it is to form surface foam. Anti-foam additives, which are mostly silicone-based, are added to the oil during production. When mixing oils containing different additives, special caution is recommended in the case of oils with significantly different silicon contents. Even if the oils are approved for the same purpose or meet the same specifications, this does not mean that they will exhibit the same foaming tendency when mixed. Even mixing an oil containing only few additives with an oil that contains many often leads to increased foam formation. In specific cases, the problem can be avoided by adding an anti-foam additive provided by the oil manufacturer. In the majority of cases, however, OELCHECK tribologists are obliged to urgently recommend a complete oil change.
Water separation
Contamination of a hydraulic oil with water is one of the most common causes of damage. Whether it enters the hydraulic system as condensate, as rain, or during the high-pressure cleaning of systems, water accelerates corrosion and cavitation. Its strong polarity and significantly contrasting density mean that water normally separates quickly from oil. Separation may however be negatively affected by additives and impurities. An excess of water – in hydraulic systems, over 500 ppm – should separate quickly from the oil, allowing it to be diverted to the deepest part of the tank so that it does not participate in oil circulation. For HLP hydraulic oils, the hydraulic oil standard specifies a maximum water separability of 30 minutes. Modern water separators then enable the water to be removed from a hydraulic tank in such a way that none of the potential forms of damage occur. For hydraulic oils used in construction or agricultural machinery, on the other hand, an opposite effect may also be desirable. The oils in these machines should not deposit the water quickly in the manner of demulsifying fluids, but should instead have a dispersing and detergent effect (HLP-D oils). These must ensure that moisture that has penetrated into the system through hydraulic cylinder movement is neutralised in microdispersed form and suspended.
Filterability
Alongside water, failures in hydraulic systems are most frequently caused by hard contaminants in the form of dust or wear particles. Hydraulic systems therefore require filters in order to function properly. These ensure that the entire system is able to operate, as well as enabling the longest possible service life for its components and oil. Modern hydraulic fluids must demonstrate good filterability. The average pore width of filter media was previously 10 to 20 μ. Today, however, the filters fitted in these systems have a pore width of 3 to 12 μ. Lab data on the filterability of an oil describe its behaviour when flowing through a filter. In the event that excessively short filter lifespans are noted following a change of oil or filter, the test results of the used oil should be compared with those of the fresh oil. In practice and in laboratory tests, the oil responsible for the problem often leaves dark, sticky deposits on the filter medium or has a low oil purity. The cause may lie in a differing additive content, or in the differing base oils of the mixed oil types. Filterability testing will ultimately provide information on the cause of the poor filterability and short filter lifespan.
Conclusion
Hydraulic fluids are miscible with one another – with the exception of PAG-based hydraulic fluids. Whether oils which the manufacturer has designated as miscible are also compatible with one another, on the other hand, can only be established by thorough laboratory testing. To this end, alongside constituent additives and base oils, such characteristics as foaming tendency, air release time, water separation, and filterability must also be examined. In the event that a problem with a hydraulic system caused by mixing incompatible oils does occur, an oil change is generally the only available solution.
OELCHECKER Summer 2018, pages 5-7